Ⅰ. 서론
인구와 산업이 도시에 집중되고 개발지역이 확대되면서 유역 환경이 크게 변화하고 있다. 유역에서의 개발지역은 불투수면의 확대로 자연적인 물순환을 왜곡시키고, 비점오염 농도를 증가시키는 등 하천의 수질과 수생태계 건강성을 저하시키는 중요한 요인으로 부각되고 있다 (Nagy et al., 2012; An et al., 2016; Hwang et al., 2016). 한편, 하천에 인접한 수변림은 유역으로부터 유입되는 퇴적물, 유기물, 영양염 등의 이동 기작에 직접적인 영향을 주는 것으로 알려져 있다 (Casotti et al., 2015; Chellaiah and Yule, 2018). 특히 수변림은 하천 제방의 침식 방지 (Pusey and Arthington, 2003), 유기물 및 영양염 저감 (Popov et al., 2006), 수온 조절 (Meek et al., 2010; Brooks et al., 2012), 수생 및 육상 생물의 서식처 제공 (Scott et al., 2009; Fernandes et al., 2011), 생태학적 건강성과 다양성 증대 (Broadmeadow and Nisbet, 2004), 토지이용이 하천에 미치는 부정적 영향 완화 (Li et al., 2009; Taniwaki et al., 2017) 등 다양한 기능을 하며, 하천의 수질 관리 및 보존 측면에서 그 중요성이 강조되고 있다. 일반적으로 하천과 인접하고 있는 수변림의 폭이 넓을수록 그 효과가 큰 것으로 알려져 있으며(Abu-Zreig, 2001; Borin et al., 2005), 환경부에서는 수계법을 제정하여 상수원 수질 보전을 위해 하천 양안 500m를 수변구역으로 지정하여 기존 수변림을 무분별한 개발로부터 보호하고, 수변구역 내 사유지를 매수하여 수목을 식재하는 등의 관리를 하고 있다.
전 세계적으로 개발지역이 확대되면서 수변림이 파편화(fragmentation) 되고 면적이 감소하고 있다 (Michalski et al., 2008; de Souza Soler et al., 2009). 하나로 구성된 수변림이 여러 개의 작은 조각으로 나누어질 때 수변림이 파편화되거나 단절되었다고 하며, 이러한 현상의 주된 원인은 인간에 의한 개발지역의 규모 확대이다 (Aguiar and Ferreira, 2005; Von Schiller et al., 2008). 수변림의 파편화는 토양 침식, 하천 수질 저하, 생물다양성 감소 등의 문제점을 야기한다 (Al-Shami et al., 2013; Collinge, 1996). 특히 하천으로 유입되는 수질오염물질의 종류와 양, 농도에 부정적 영향을 주고 (Shen et al., 2015), 강우유출수의 유속과 유량 증가, 수변림 내 체류시간 감소 등 수문학적 특성의 변화로 수변림의 퇴적, 침투, 흡수, 흡착, 여과, 증발산과 같은 기작의 효율이 저하되어 결과적으로 하천 유기물, 영양염, 탁도 증가, 침전물로 인한 기질 변화 등으로 하천 수질에 부정적인 영향을 미치게 된다 (Meave et al., 1991; He et al., 2000). 선행연구에 의하면 수변림의 공간적 구조(spatial pattern)는 유기물의 분해 효율과 질소 및 부유 물질 농도 등에 영향을 주는 것으로 나타났으며 (de Souza et al., 2013; Fernandes et al., 2014), 수변림의 공간적 구조 변화는 유역 및 하천의 수문학적 특성 변화를 야기하게 되고, 결과적으로 하천 수질에 영향을 주게 된다(Shandas and Alberti, 2009; Gonzales-Inca et al., 2015).
유역의 토지이용과 하천 수질과의 관계를 통계적 방법론으로 분석한 연구들은 주로 상관분석, 단순회귀분석 또는 다중회귀분석 등 OLS(ordinary least square)에 기반하였다 (Lee et al., 2009; Park et al., 2011; Clapcott et al., 2012; Shen et al., 2015). 이러한 방법론은 다수의 독립변수와 종속변수가 존재할 경우 인과관계 분석이 어려우며, 종속변수에 대한 독립변수들의 구조적 관계를 분석할 수 없다는 한계를 가진다. 이러한 한계를 보완하기 위해 구조적 인과관계를 분석할 수 있는 구조방정식모형(SEM, structural equation model)이 여러 분야에서 널리 적용되고 있다. 구조방정식모형은 국내외적으로 주로 사회과학 분야의 연구에서 활용되었으나, 최근 자연과학 분야에도 적용되고 있다 (Lee et al., 2013; Kim and Lee, 2018; Kim et al., 2018). 구조방정식 모형은 확인적 요인분석(confirmative factor analysis)과 경로분석(path analysis)을 결합하여 경험적 인과관계를 분석할 수 있도록 개발되었다. 구조방정식 모형을 통해 변수들 간 관계를 동시에 추정할 수 있으며, 측정오차를 제시하기 때문에 신뢰도 확보도 가능하다는 장점이 있다 (Kim, 2009; Kim and Lee, 2018).
하천의 수질은 단일 요인에 의해 영향을 받는 것이 아니라 여러 요인들이 상호작용하는 인과관계에 의해 영향을 받는다. 본 연구는 구조방정식모형(SEM, Structural Equation Model)을 이용하여 하천 내 총질소(TN: Total Nitrogen) 농도에 대한 하천차수, 고도, 경사를 포함하는 하천환경 특성과 유역의 개발지역 면적, 수변림의 파편화 정도의 구조적 영향 분석에 목적을 두고 수행되었다. 본 연구의 결과는 수질에 미치는 영향을 최소화하기 위한 유역의 토지이용, 그리고 하천 수질 보전 및 관리를 위한 효율적인 수변구역 관리에 직접적으로 활용될 수 있다.
Ⅱ. 연구방법
한반도 남동부에 있는 낙동강 대권역은 32개 중권역과 267개 소권역으로 구성되어 있다 (Figure 1 참조). 낙동강 대권역의 유역면적은 31,314km2, 유로 연장은 699.91km이며, 국가하천 16개와 지방하천 879개를 포함하고 있다 (Ministry of Environment, 2014). 낙동강 본류는 강원도 태백산에서 발원하여 낙동강 유역 중심부를 관류하며, 경상북도와 경상남도를 지나 남해로 유입된다. 주요 지류로는 상류의 내성천, 미천, 중류의 금호강, 황강, 하류의 남강, 양산천 등이 있다. 연평균 강수량은 전국 1,274mm의 90% 수준이며, 연평균 기온은 유역의 상류인 강원 지역의 경우 10°C, 남부 지역으로 갈수록 점차 높아져 부산의 경우 15 °C로 나타났다 (https://www.busan.go.kr/environment/ahnakdonggang02). 낙동강 유역은 백두대간으로 둘러싸여 분지를 형성하며, 대륙성 기후의 특성이 강하다. 낙동강 상류 유역은 산지가 많으나, 전반적으로 경사도가 완만하며 낙동강 본류는 안동, 구미, 부산, 대구 등의 주요 대도시와 산업·공업 단지, 농촌 지역 등의 입지로 토지이용으로 인한 오염 부하량이 높고, 인구는 2014년 기준으로 13,293천 명이며, 2010년부터 지속적으로 증가했다. 특히 낙동강 수계는 하천환경뿐만 아니라, 유역 토지이용의 지속적인 증가와 4대강 사업 등에 의한 수변림의 구조 변화 등이 뚜렷하고, 환경부의 재자연화 사업, 지류지천 살리기 사업 등의 복원 대상지를 다수 포함하고 있어 연구결과의 활용성이 높을 것으로 판단된다 (Ministry of Environment, 2014).
본 연구에서는 총질소를 수질 항목으로 선정하였다. 영양염류 농도를 기준으로 하는 총질소 농도는 하천의 오염 정도를 측정하는 대표적인 수질 지표 중 하나이다. 환경부에서 전국 하천을 대상으로 실시하고 있는 「하천 수생태계 현황 조사 및 건강성 평가」 연구에서는 2007년부터 매년 부착돌말류, 저서성 대형무척추동물, 어류 등 수생 생물군의 건강성과 하천의 수질, 수리·수문, 서식수변 환경 등의 하천환경 특성을 모니터링해오고 있으며, 각 지점에 대한 조사는 봄과 가을 연 2회 실시하고 있다. 본 연구에서 총질소 농도 값은 2012년 측정된 낙동강 대권역 「수생태계 현황 조사 및 건강성 평가 결과」의 수질 자료를 이용하였다. 수변림의 범위는 환경부 수계법에 따라 하천경계로부터 양안 500m 이내로 하였으며, 전체 250개 조사지점 중 하천 양안 500m 내 수변림(산림 및 초지)이 존재하지 않는 지점을 제외한 88개 지점을 대상으로 분석을 수행하였다.
하천차수는 하천차수도(WAMIS, 2011), 고도 및 경사는 국토지리정보원(NGII, 2014)에서 제작한 수치고도모형(DEM, Digital Elevation Model), 유역의 개발지역 면적은 토지피복도(WAMIS, 2011~2013) 세분류 자료를 이용하여 ArcMap 10.6.1(ESRI, 2018)을 통해 추출하였으며, 환경부의 권역관리계획상 소유역을 공간적 연구단위로 사용하였다. 환경부에서는 토지피복을 시가화건조지역, 농업지역, 산림지역, 초지, 습지, 나지, 수역의 총 7개의 대분류와 22개의 중분류, 41개 세분류로 구분하고 있다. 본 연구에서는 토지피복도 세분류에서 시가화 건조지역(주거, 공업, 상업 지역 등)과 농업지역(논, 밭 등)을 개발지역으로 재분류하여 수질 모니터링 지점별 개발지역 면적 비율을 계산하였다.
경관의 공간적 구조는 면적, 밀도, 가장자리(area, density, edge metrics), 모양(shape metrics), 인접도(isolation, proximity metrics), 연결성(connectivity metrics) 등의 경관 지수를 통해 분석할 수 있으며, 일반적으로 하나의 지수보다는 여러 지수를 사용하여 공간 구조를 평가한다 (Jaeger, 2000; Wang et al., 2014). 본 연구에서는 수변림의 공간적 구조를 정량적으로 분석하기 위해 기존 연구에서 사용된 지수인 PLAND (경관백분율, Proportion of Landscape), LPI (최대패치지수, Largest Patch Index), LDI (경관분할지수, Landscape Division Index), LSI (경관형태지수, Landscape Shape Index)를 선정하였다 (Table 1 참조) (e.g., Rutledge, 2003; Wang et al., 2014). ArcMap 10.6.1에서 수변지역 경계와 2012년 환경부의 토지피복도(산림 및 초지, 중분류)를 중첩하여 수변림을 최종적으로 추출하였다. 벡터 형태의 수변림 지도를 래스터 자료(10m resolution)로 변환하여 FRAGSTATS (McGarigal, 2014)에서 경관 지수를 산출하였다. 수변림은 일반적으로 중심지 면적이 넓고 가장자리 면적이 좁으며 파편화되지 않을수록 구조적으로 안정적인 것으로 판단할 수 있다. 즉, 수변림의 PLAND, LPI는 클수록, LDI, LSI는 작을수록 수변림의 파편화 정도가 낮은 것으로 판단할 수 있다(Yirigui et al., 2019).
n=number of riparian vegetation patch, ai=area of riparian vegetation patch i, e=total length of perimeter of class i in terms of number of cell surfaces and A=total area of riparian buffer zone.
구조방정식모형(SEM)은 요인분석과 경로분석이 결합된 형태로, 다수의 독립변수와 종속변수 간 인과관계를 동시에 추정할 수 있으며, 특히 데이터 분석결과를 시각화 하여 결과물의 해석이 용이하다는 장점이 있다 (Kim, 2009; Kim and Lee, 2018). 추정된 모형은 크게 잠재변수, 관측변수, 오차로 구성되며, 직접적인 관측 가능 여부에 따라 잠재변수와 측정변수로 분류한다. 일반적으로 가설적 연구모형을 설정하고, 상관관계 분석을 통해 중요도가 낮은 변수를 제거하여 타당성을 확보하며, 최종 선정된 변수를 이용하여 경로도형을 구축한 후 모형을 추정한다. 추정된 모형의 χ2, GFI(the goodness-of-fit index), AGFI(adjusted goodness-of-fit index), RMSEA(root mean square error of approximation residuals), NFI(normed fit index) 등의 지수를 통해 모형의 적합도(goodness-of-fit)를 평가하고, 모형이 적합할 경우 인과관계를 판단한다. 본 연구에서는 하천환경 특성(하천차수, 고도, 경사), 유역의 개발지역 면적, 수변림의 공간적 구조가 하천의 총질소 농도에 영향을 미칠 것이라는 가설적 연구모형을 설정하고, 모형 추정, 평가 및 수정 과정을 통해 모형 설명력이 우수한 변수들을 이용하여 단계적으로 최종 구조방정식 모형을 추정하는 접근방법을 채택하였으며, 모형 추정은 IBM SPSS Amos 25 프로그램을 이용하였다.
Ⅲ. 결과 및 고찰
하천환경 특성, 개발지역 면적 비율, 수변림의 공간적 구조, 수질의 기초 통계량은 Table 2와 같다. 하천환경 특성 중 하천차수는 1차부터 7차까지 분포하고, 평균 고도는 80.68m로 우리나라 평균 고도(482m)에 비해 낮으며, 평균 경사는 13.68%로 나타났다. 특히, 고도의 표준편차 값이 높게 나타나 유역별 차이가 큰 것으로 나타났다. 개발지역 면적 비율의 평균은 31.03%로 나타났으며, 수변림의 공간적 구조 지수인 PLAND, LPI, LDI, LSI의 평균값은 각각 35.37, 20.73, 0.92, 1.85로 나타났다. 수질 측정 항목인 총질소 농도는 평균값이 2.77 mg/L로, 「환경정책기본법」 시행령에 제시된 호소수 수질환경기준에 따르면 5등급에 해당하여 전반적으로 수질이 불량한 것으로 나타났다.
n = 88.
본 연구에서 사용된 변수들의 상관관계를 분석한 결과, 하천 내 총질소 농도는 하천환경 변수나 수변림의 경관 지수와 직접적인 상관관계를 보이지는 않는 것으로 나타났다 (Figure 2 참조). 총질소 농도는 유역의 개발지역 면적과 양의 관계를 갖는 것으로 나타났으며(r=0.34), 이는 개발지역 비율이 높을수록 하천 총질소 농도가 높은 것을 의미한다. 하천환경 변수 중 하천차수는 LDI(r=0.21)를 제외하고는 유의한 상관관계를 보여주지 않았으나, 고도와 경사는 대부분의 수변림 경관 지수, 개발지역 면적과 유의한 상관관계를 갖는 것으로 나타났다. 구체적으로 고도는 PLAND(r=0.50), LPI(r=0.30), LDI(r=−0.35), 그리고 개발지역 면적(r=−0.39)과 유의한 상관관계를 보여주었다. 이러한 경향은 하천 경사와 수변림의 경관 지수, 개발지역 면적의 관계에서도 매우 유사하게 나타났다. 즉, 고도가 높고 경사가 급할수록 수변림의 파편화 정도와 개발지역 면적이 낮은 것으로 해석된다.
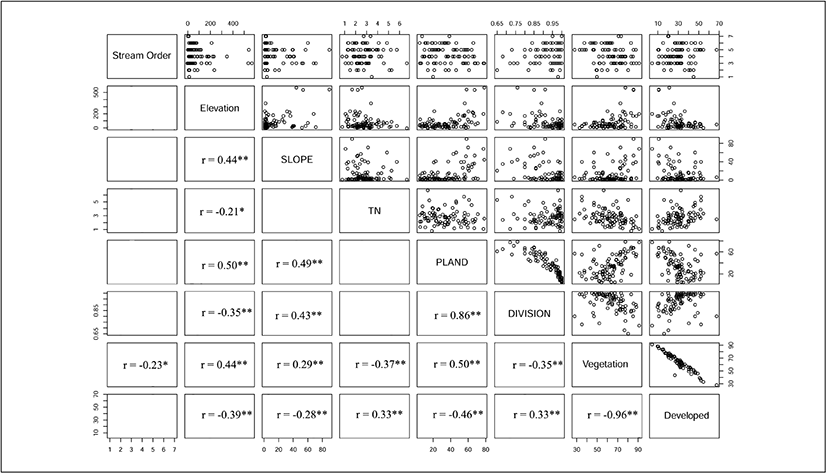
수변림의 경관 지수 중 PLAND는 다른 경관 지수 및 개발지역 면적(r=−0.44)과 유의한 상관관계를 보이며 개발지역 면적이 높을수록 수변림의 파편화 정도가 높은 것을 의미한다. 다만 수변림 형태 지수인 LSI는 PLAND와만 유의한 상관관계로(r=0.20) 파편화 정도가 높을수록 수변림이 복잡한 형태인 것으로 해석할 수 있다.
개발지역 면적은 하천차수와 LSI를 제외한 모든 변수와 유의한 상관관계를 갖는 것으로 나타났다. 구체적으로 개발지역 면적은 총질소 농도(r=0.34)와 양의 상관관계, 고도(r=−0.39), 경사(r=−0.27), PLAND (r=−0.44), LPI(r=−0.32), 그리고 LDI(r=−0.32)와 음의 상관관계를 갖는 것으로 나타났다. 이는 높은 고도와 급경사 지역에서는 개발지역 면적이 낮고, 개발지역 면적이 높은 경우 수변림의 파편화 정도가 높은 것으로 해석된다. 상관관계 분석결과는 변수들의 인과관계 파악은 어려우나, 고도가 높고 경사가 급한 지역에서는 개발이 어렵거나, 개발비용이 높아 개발지역 면적이 낮고, 개발이 적은 지역은 수변림의 파편화 정도가 낮은 것으로 해석할 수 있다. 즉, 하천환경 특성이 개발지역 면적을 결정하고, 개발지역 면적은 수변림의 파편화 정도를 결정하는 것으로 추정할 수 있다. 따라서 구조방정식에서 하천환경 특성에 의한 개발지역 면적의 모형을 우선으로 추정하였다.
고도, 개발지역 면적, 수변림 공간적 구조를 이용한 총질소 농도 모형을 도출하기 위해 총 세 단계의 가설적 구조방정식 모형을 설정하였다. 첫 번째 단계에서는 상관관계 분석결과에 기초하여 하천차수, 고도, 경사를 독립변수로, 유역의 개발지역 면적을 종속변수로 하는 가설적 구조방정식 모형을 설정하였다. 모형을 추정한 결과, 하천차수와 경사는 유의하지 않은 것으로 나타났으며, 고도는 높을수록 개발지역 면적이 감소하는 것으로 나타났다 (Figure 3a 참조). 고도가 높은 지역의 경우, 개발이 제한되어 있거나 접근성이 떨어져 개발되지 않고 보전된 경우가 많아 고도가 낮은 지역일수록 개발지역의 면적이 넓은 것으로 판단된다 (Ye et al., 2009; Chen and Lu, 2014). 두 번째 단계에서는 위 단계에서 추정된 모형에 하천 내 총질소 농도를 추가하여 고도는 하천의 총질소 농도에 직접적인, 그리고 개발지역 면적을 통한 간접적인 영향을 모두 갖는 것으로 설정하였다. 고도의 총질소 농도에 대한 직접적인 영향은 통계적으로 유의하지 않은 것으로 나타났으나, 고도는 개발지역 면적을 낮추고(β=−0.39), 개발지역 면적은 하천 내 총질소 농도를 높이는(β=0.34) 것으로 나타났다 (Figure 3b 참조). 즉, 고도는 직접적으로 수질에 영향을 미치지는 않으나 (Park et al., 2019), 유역의 개발지역 면적을 통해 간접적으로 수질에 영향을 미치는 것으로 볼 수 있다. 선행연구에 의하면 유역 내 도시지역이 많을수록 불투수층 면적이 확대되며, 강우유출 특성과 비점오염물질 거동의 변화로 하천 수질이 악화되고 (Carpenter et al., 1998; Shen et al., 2015), 농경지는 비료와 살충제 사용으로 하천 내 총질소를 포함한 유기물, 영양염 농도를 증가시키는 주요 원인으로 알려져 있다 (Woli et al., 2004).
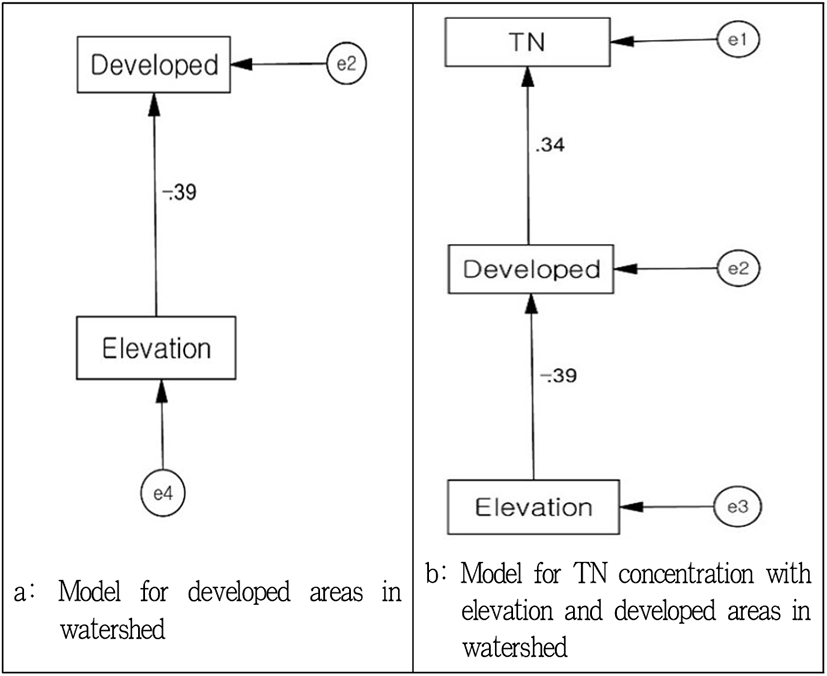
Figure 3b 모형을 기초로 수변림 경관 지수(PLAND, LPI, LDI, LSI)를 모형에 추가하여 세 번째 단계인 최종 가설적 모형을 설정하고, 유의하지 않은 경로를 제외한 최종 모형을 추정하였다 (Figure 4, Table 3 참조). 고도는 개발지역 면적에 부정적 영향, PLAND에 긍정적 영향을 주는 것으로 나타나(β=−0.39, β=0.24), 고도가 높아지면 개발 면적이 낮고, 수변림의 파편화 정도가 낮은 것으로 해석할 수 있다. 개발지역 면적은 LDI에 긍정적인 영향을(β=0.32), 그리고 LPI(β=−0.32)와 PLAND(β=−0.44)에 부정적인 영향을 주는 것으로 분석되었다. 이는 유역의 개발지역 면적이 높을수록 수변림의 파편화가 심해지는 것으로 해석할 수 있다. LSI를 제외한 수변림의 공간적 구조는 하천 내 총질소 농도와 유의한 관계를 갖는 것으로 나타났다. 구체적으로 PLAND(β=−0.31)와 LDI(β=−0.31)는 총질소의 농도를 낮추고, LPI는 총질소의 농도를 높이는 것으로 나타났다(β=0.25).
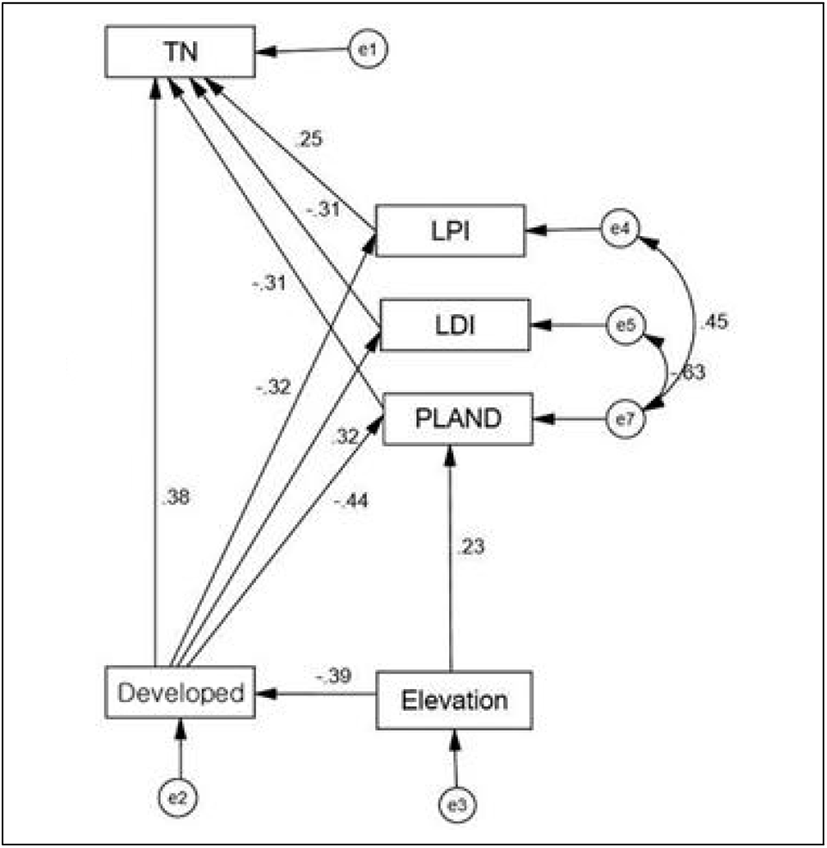
추정된 최종 모형 Figure 4의 적합도 검증 결과, RMSEA와 PNFI를 제외한 나머지 평가지표들을 모두 만족하는 것으로 나타나 (Table 4 참조), 최종 모형이 고도, 유역의 개발지역 면적, 수변림의 공간적 구조가 하천의 총질소 농도에 미치는 영향을 적합하게 설명하는 것으로 나타났다.
최종 모형에 의하면 개발지역 면적은 총질소 농도에 직접적인 영향을 주는 것으로 나타났다. 도시지역이나 농경지와 같은 개발지역이 수질에 미치는 부정적인 영향은 기존의 많은 연구를 통해 검증되었다 (Osborne and Wiley, 1988; Woli et al., 2004; Baker, 2006; Chang, 2008; Uriarte et al., 2011; Wan et al., 2014; Ding et al., 2016; Huang et al., 2016). 또한, 개발지역 면적은 수변림의 공간적 구조를 통해 총질소 농도에 간접적으로도 영향을 주는 것으로 나타났다. 개발지역 면적과 수변림 파편화의 관계는 선행연구를 통해 지속적으로 보고되었으며 (Allan, 2004; Ferreira et al., 2005; Von Schiller et al., 2008), 유역 토지이용이 수질에 미치는 부정적 효과는 수변림과 수변림의 공간적 구조에 의해 완화될 수 있는 것으로 나타났다 (Gharabaghi et al., 2002; Shandas and Alberti, 2009; Fernandes et al., 2011; Gonzales-Inca et al., 2015; Yirigui et al., 2019). 수변림은 강우유출수의 체류시간을 증가시키고, 영양물질, 퇴적물, 유기물을 여과, 흡착함으로써 개발지로부터의 오염물질을 제거하여 하천 수질에 대한 영향을 완화하며 (Meave et al., 1991; He et al., 2000), 특히 수변림의 공간적 구조는 질소, 부유 물질의 농도와 유기물의 분해에 영향을 주는 것으로 보고되었다 (de Souza et al., 2013; Tanaka et al., 2014). 즉, 수변림의 공간적 구조에 따라 그 효과는 달라지며, 수변림이 공간적으로 파편화 될수록 하천의 수질에 부정적인 영향을 주게 된다 (Shandas and Alberti, 2009; Gonzales-Inca et al., 2015).
Ⅳ. 결론
하천의 수질은 다양한 요인들의 인과관계에 의해 영향을 받는다. 본 연구에서는 낙동강 수계를 대상으로 하천환경 특성, 유역의 개발지역 면적, 수변림의 공간적 구조가 하천의 총질소 농도에 미치는 영향을 구조방정식모형을 이용하여 체계적인 인과모형으로 제시하고 검증하였다. 가설적 모형을 토대로 경로 도형을 설정하여 모형을 확인하고, 수정을 통해 최적의 모형을 선택하였으며, 추정된 모형의 적합도를 도출하였다. 최종 모형에서 고도가 낮을수록 개발지역 면적은 증가하고, 개발지역 면적의 증가는 하천의 총질소 농도를 높이는 것으로 나타났다. 또한, 개발지역 면적의 증가는 수변림의 파편화 정도를 높이고, 결과적으로 하천의 총질소 농도에 영향을 주게 된다. 즉, 개발지역은 고도에 의해 한정되고 수변림의 공간적 구조는 개발지역 면적에 의해 결정되며, 개발지역 면적은 총질소 농도에 직접적인 영향과 수변림의 공간적 구조를 통해 간접적으로 영향을 주는 것으로 나타났다.
통계적 방법론을 이용하여 하천차수, 지형 등의 하천환경 특성, 유역의 토지이용, 수변림의 유무 등이 하천의 수질에 미치는 영향을 분석한 기존 연구들은 인과관계 분석, 즉 종속변수에 대한 독립변수들의 구조적인 관계를 분석할 수 없다는 한계가 있었다. 즉, 하천차수와 수질과의 관계, 유역의 토지이용과 수질과의 관계 등에 대한 분석은 가능하였으나, 하천차수, 지형, 유역의 토지이용, 수변림이 어떠한 인과관계를 통해 하천의 수질에 영향을 미치는가에 대한 분석은 기존의 방법론을 통해서는 도출하기 어려웠다. 본 연구에서는 하천환경 특성, 유역의 개발지역 면적, 수변림의 공간적 구조와 하천의 수질 간 구조적인 관계에 초점을 두고 수행되었다는 측면에서 의의가 있다. 본 연구의 결과에 의하면 유역의 개발지역은 하천의 총질소 농도에 직간접적으로 영향을 미치나, 수변림이 공간적으로 안정적인 구조를 갖고 식생의 수량이 풍부할 경우 개발지역이 하천 수질에 미치는 부정적인 영향을 상당히 완화시킬 수 있을 것으로 판단된다. 유역의 개발지역을 최소화하고 수변림의 양적 확보와 구조를 복원하는 것이 하천의 수질 회복에서 중요함을 시사한다.
그러나 본 연구는 낙동강 수계만을 대상으로 제한된 변수들을 사용하여 분석하였기 때문에 연구결과를 일반화하는 것은 무리가 있다. 연구 대상지의 확대와 하천 수질에 영향을 미치는 다양한 종속변수와 수질 측정 항목, 생물지수까지 포함한 구조방정식모형을 도출하는 후속 연구가 요구된다. 특히 본 연구에서 수질 지수로 선정한 총질소 농도는 농업용 비료, 가축 분뇨, 미처리된 하수나 공장 폐수 등에 가장 영향을 많이 받으므로 향후 이러한 변수들을 포함하는 구조적인 관계에 대한 연구가 필요할 것으로 판단된다.