1. Introduction
Thermal comfort is crucial for human well-being outdoors (Migliari et al., 2022). Extreme heat events can lead to increased morbidity and mortality (Song et al., 2021), impacting mental health and discouraging outdoor activities on hot days (Hansen et al., 2008). Improving outdoor thermal conditions involves implementing strategies such as increasing shade coverage and expanding green spaces (Phelan et al., 2015). Shade provision directly mitigates thermal discomfort by reducing direct shortwave radiation fluxes and lowering the temperature of shaded surfaces (Martinelli et al., 2015). Additionally, it decreases longwave radiation fluxes, further enhancing the surrounding thermal environment. Concurrently, augmenting green spaces in urban areas stands as a widely acknowledged method for ameliorating outdoor thermal comfort, achieved through the absorption of solar radiation and evapotranspiration (Taleghani, 2018). Nevertheless, the challenge of limited land availability in urban settings often renders the expansion of green spaces impractical, leading to the exploration of alternative solutions such as green walls and roofs (Venter et al., 2020).
To evaluate the efficacy of these strategies, research often employs computational models like the predicted mean vote (PMV), physiological equivalent temperature (PET), and universal thermal climate index (UTCI), alongside human surveys (Martinelli et al., 2015). However, these calculations may not fully encapsulate the nuanced perceptions of individuals in real-world thermal conditions. Recent studies advocate for a more comprehensive approach, integrating physiological measures such as skin temperature, heart rate variability (HRV), electrocardiogram (ECG), and electroencephalogram (EEG) to better understand thermal comfort (Lin et al., 2020; Han and Chun, 2021). EEG, in particular, offers insights into direct nervous system responses, complementing psychological indices obtained from surveys (Son and Chun, 2018). This holistic approach acknowledges the multidimensional nature of thermal comfort, encompassing both physiological sensations and psychological perceptions (Wang and Liu, 2020). Consequently, it provides a more nuanced understanding of how environmental interventions impact human experiences and well-being.
Recent research has uncovered a significant correlation between specific brain wave frequencies and the emotions individuals experience, particularly in response to changes in thermal conditions. Han and Chun (2021) conducted EEG analysis to decipher thermal displeasure, revealing a decrease in theta power alongside increased beta and gamma powers during discomfort induced by heat. Similarly, Zhu et al. (2020) observed heightened beta power and diminished theta and delta power during thermal discomfort. Lang et al. (2022) explored cognitive performance variance in diverse indoor environments, linking EEG signal power to both thermal comfort and neurobehavioral test performance. Pan et al. (2023) investigated shifts in EEG signals in response to changing indoor temperatures. However, most EEG studies have been confined indoors due to the challenges of controlling outdoor microclimates, where thermal comfort is shaped by a complex interplay of stimuli and non-thermal factors (Manavvi and Rajasekar, 2023). Recognizing the distinct expectations for thermal comfort indoors versus outdoors (Höppe, 2002), there’s a pressing need for research centered on outdoor contexts. While prior studies have highlighted the efficacy of greenery systems for improving outdoor thermal conditions, they lack integration of physiological and psychological data within real-world outdoor experiments. Furthermore, the outdoor application of EEG for thermal comfort assessment remains largely unexplored, leaving a critical gap in understanding how such interventions influence human experiences.
Against this backdrop, this research aims to investigate the effects of landscape amenities on subjective human thermal comfort physiologically, using EEG, and psychologically, using a questionnaire survey. We conducted an experiment on the rooftop of a four-story public building in Gwunsun-gu, Suwon city, South Korea, on September 4–8, 2021. Three types of greenery systems were investigated: facing a green wall, sitting against a green wall, and viewing a green roof. These were compared with two conditions; baseline and being under a shade canopy. The baseline represents a setting without any landscape amenities, while the spatial setting with a shade canopy serves as the benchmark, employing the most direct measures to mitigate heat. This is the first attempt to evaluate outdoor landscape amenities in terms of thermal comfort through a human experiment using EEG and a questionnaire survey.
2. Analysis Design
The study site was the rooftop of the Suwon Urban Development Corporation, a four-story public building, located in Gwunsun-gu, Suwon city, Seoul Metropolitan Area, South Korea (Figure 1). Surrounding buildings were shorter, preventing shadow casting. Over the past decade (2011-2021), the area has maintained an average air temperature of 25.06°C and received an average summer precipitation of 245.13mm. Due to an unusually prolonged rainy season in the summer of 2021, the experiment took place from September 4–8 to ensure sufficiently high ambient temperatures, conducted between 10 am and 6 pm.
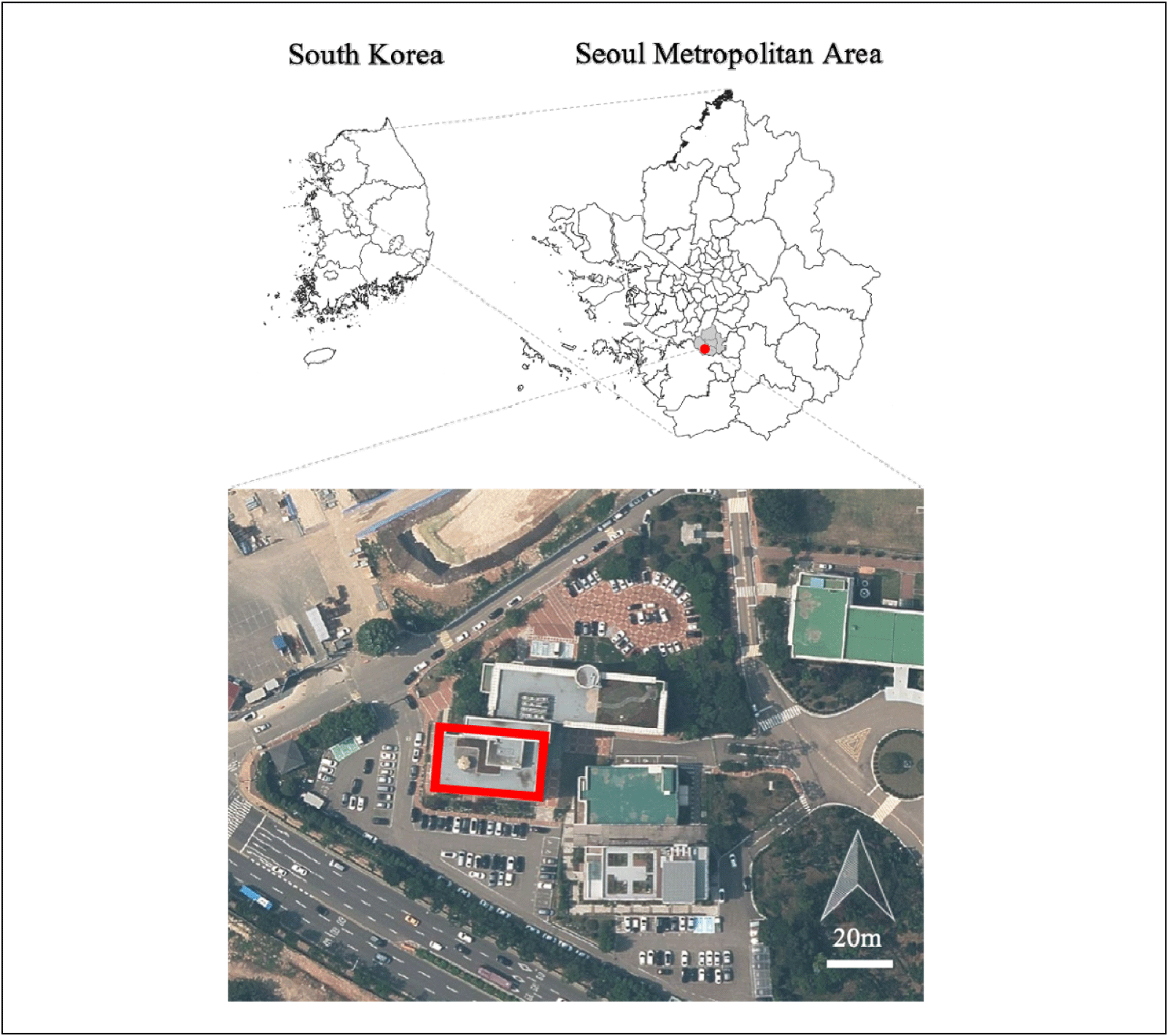
In this experiment, we examined the impact of landscape amenities, such as green walls, green roofs, and shade canopies, on thermal comfort levels across five distinct spatial settings. Participants were positioned to face the green wall, sit against it, sit under the shade canopy, and observe the green roof units, with the absence of any such structures serving as the reference point for the lowest thermal comfort level. Sitting under the shade canopy was deemed the most comfortable setting due to direct radiation blockage. Considering the psychological effects of viewing greenery reported in previous studies (Velarde et al., 2007), we included two variations with the green wall: facing and sitting against it.
The five spatial arrangements were depicted in Figure 2. The green wall measured 2,200 × 2,300 mm and featured Hedera helix and Pachysandra terminalis plants, while the green roof, planted with Sedum takesimense Nakai, measured 1,800 × 1,600mm. Each zone was separated by at least 2m to minimize interference between experiments. While randomization was necessary, we maintained a consistent measurement order for each setting to preserve distances and minimize interference. Furthermore, to ensure uniform sunlight exposure, all spatial arrangements faced the same direction. The spatial settings maintained consistent levels of sunlight exposure and ambient noise across all setups to ensure comparability. However, as the experiment was conducted outdoors, naturally occurring noises, such as airplanes, were not controlled. These environmental factors were acknowledged as inherent characteristics of outdoor experiments.
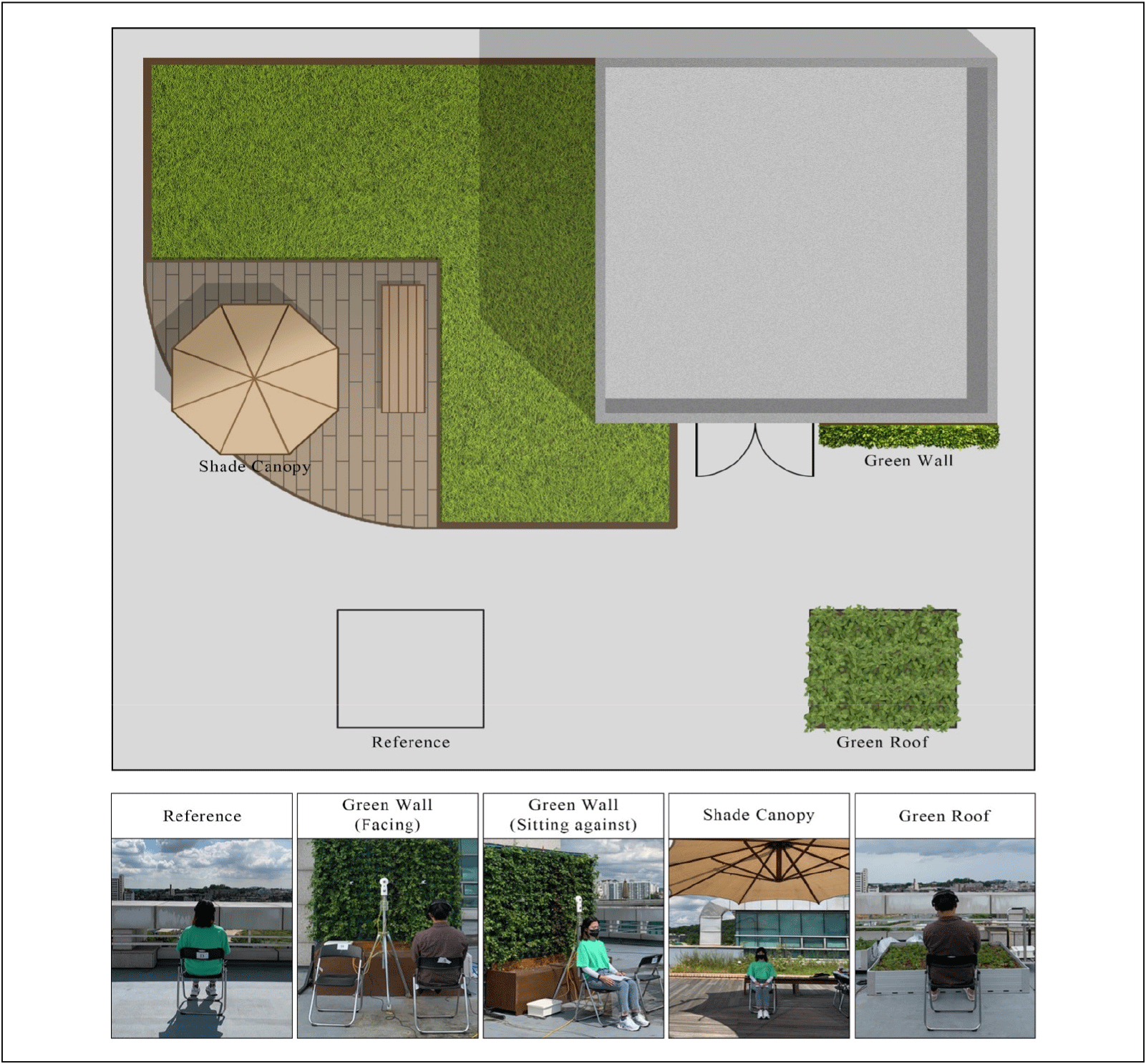
In our study, we gathered data through questionnaire responses and EEG measurements from a cohort of 57 healthy adults without underlying health conditions. To mitigate the risk of heat-related ailments, we limited each measurement to three minutes. Participants rotated through each setting, spending three minutes, including 15 seconds of meditation with eyes closed, before moving to the next without breaks, as illustrated in Figure 3. We carefully designed the experimental sequence to minimize any potential bias caused by the order of exposure to different settings. Specifically, the reference condition was strategically placed between the green wall and green roof measurements, ensuring it was measured mid-experiment rather than at the start or end. This arrangement allowed for a balanced assessment of the reference condition, reducing the potential carryover effects from exposure to either greenery system.
The questionnaire comprised two sections: personal data and subjective assessments of thermal comfort in each setting. The personal data section included details such as body mass index (BMI), underlying health conditions, age, sex, physical and mental state, pre-experiment activities, and transportation mode. The second section utilized the thermal sensation vote (TSV) and thermal preference vote (TPV) from ASHRAE 55 to evaluate participants’ thermal comfort levels. The thermal sensation vote (TSV) and thermal preference vote (TPV) surveys utilized in this study are based on the methodology outlined in ASHRAE Standard 55, which provides widely recognized guidelines for assessing thermal comfort (ASHRAE, 2020). While this standard primarily addresses indoor environments, its principles, including the use of the Predicted Mean Vote (PMV) and Predicted Percentage of Dissatisfied (PPD) models, have been effectively adapted in outdoor thermal comfort research. TSV and TPV, commonly employed in thermal environment questionnaires, were specified by body part and humidity levels. As TPV is on a three-point scale, it was rescaled to match the seven-point scale of TSV. Smaller TPV values indicated higher preferences for warmer conditions, while larger values suggested a preference for cooler temperatures.
EEG data were collected using the EPOC X multi-channel EEG device by Emotiv Inc. USA, featuring 14 sensors positioned on the scalp based on the international 10–20 system. The collected EEG data were transmitted wirelessly to a computer for analysis using Bluetooth. The analysis focused on six emotional indices provided by the manufacturer: engagement, excitement, focus, interest, relaxation, and stress. These indices, ranging from 0 to 1, have demonstrated reliability and validity in previous studies (Lin et al., 2020), with each index recorded simultaneously. This study was approved by the ethics committee of Seoul National University (IRB No. 2106/002-021).
We employed a fixed-effects multilevel regression model to analyze within-subject variations across five spatial settings. The primary aim of this study was to evaluate how specific environmental conditions influenced EEG outcomes and thermal perceptions, which were modeled as fixed effects. This approach allowed us to directly assess the impact of these controlled settings. Participant-specific characteristics, including BMI, age, gender, and transportation mode, were included as random effects in the multilevel model to account for participant-level variability while minimizing overfitting. This approach aligns with the primary focus of this study, which is to evaluate the influence of environmental conditions on EEG outcomes and thermal perceptions, rather than the direct effects of participant-specific characteristics. By treating these characteristics as random effects, we were able to control for individual differences while maintaining the focus on key independent variables, such as spatial setting and condition. The multilevel model structure addressed the inherent correlations within repeated measures, effectively accounting for dependencies within participants while controlling for group-level characteristics (Jung et al., 2016). In our analysis, individual observations were specified as Level 1, nested within participants at Level 2, enabling a robust examination of thermal comfort indices across experimental conditions. The model is presented below as Function (1):
Level 1:
Level 2:
Our dependent variables were the responses from the participants and the psychological and physiological indices. The psychological indices consisted of the thermal sensation vote (TSV) and thermal preference vote (TPV). All questionnaires related to the thermal environment were scored on a seven-point scale. Because we considered the EEG and the two psychological indices, we ran the regression multiple times. The dependent EEG variables consisted of six emotion indices, and the TSV results are categorized by part of the body and humidity (Table 1). Spatial settings with landscape amenities served as question variables: facing the green wall (Greenwall_front), sitting against it (Greenwall_back), under the shade canopy (Shade_canopy), looking at the green roof (Greenroof), and an empty space designated as the reference (Reference). Individual characteristics were considered controls, including sex (Male, Female), age categories (20s, 30s, 40s), and body mass index (BMI) categories (Underweight, Normal, Overweight, Obese, Extreme obese). Pre-experiment activities were categorized as indoor (Indoor) or outdoor (Outdoor), while transportation mode was classified as car (By_car), public transportation (By_public), or walking (By_walk). Physical and mental conditions on the experiment day were categorized as worse than usual (Worse), usual (Usual), or better than usual (Better).
3. Results
Among the 57 participants, the sex ratio was almost equal (47.368% female, 52.632% male). The average age was 27.018 years, with the majority in their 20s (75.439%), followed by their 30s (22.807%) and 40s (1.754%). Less than half had a normal BMI (18.5–23, 43.860%), while others were overweight (23–25, 22.807%), obese (25–30, 19.298%), underweight (under 18.5, 10.526%), or extremely obese (over 30, 3.509%). Indoor activity (61.404%) was more common than outdoor activity (38.596%) before the experiment. Public transportation (43.860%), private cars (40.351%), and walking (15.789%) were the most common transportation modes to the experimental site. Participants’ overall condition on the experiment day was typically usual (75.439%), with some feeling worse (12.281%) or better than usual (12.281%).
The multilevel analysis indicates that landscape amenities designed to enhance the outdoor thermal environment significantly influence users’ physiological and psychological well-being (Tables 2 and 3). Regarding psychological status, participants perceived thermal comfort in the following order: shade canopy, facing the green wall, looking at the green roof, sitting against the green wall, and baseline. Detailed results are shown in Table 1. TSV favored zones with landscape amenities installed over the reference space across all body categories, except when sitting against the green wall. Similar trends were observed for TPV. Consistent with prior research, the shade canopy emerged as the most preferred landscape amenity for thermal comfort. Participants rated all questionnaire items most positively under the shade canopy, indicating a preference and greater thermal comfort compared to other settings. This suggests that shading structure is the most effective outdoor furnishing for enhancing thermal conditions, likely due to their direct blocking of shortwave radiation (Lin et al., 2013).
The random effects of personal traits on the questionnaire survey (Table 2) were not significant, whereas they were significant for EEG in some cases (Table 3), despite the irregular behavior of random effects across different EEG types. Therefore, it is difficult to extract clear random effects of personal traits on the questionnaire survey and EEG types in this study.
The facing-green-wall option was rated as the most thermally pleasant among the three greenery system settings. Participants reported more positive thermal sensations across whole body parts when facing the green wall compared to the reference environment. TPV results aligned with these findings. However, the difference in thermal sensation related to humidity was not statistically significant. Although previous studies have claimed that adding greenery could increase relative humidity (Jaafar et al., 2022), the participants did not feel a difference in the humidity-related comfort level from that of the reference space. This discrepancy might be due to people in warm and humid climates being less sensitive to humidity changes (Kong et al., 2019). Nonetheless, the thermal comfort provided by the facing-green-wall option was still lower than that provided by the shade canopy. When looking at the green roof, participants rated all TSV measures and TPV higher than those of the reference, except for the humidity-related question. However, the overall magnitude was smaller compared to the green wall. This difference could be attributed to the eye-level view. Vertical greening in front of participants could be predominantly seen at eye level, while green roof units below eye level might not be perceived as clearly as vertical greening. Sitting against the green wall did not show any improvement in thermal conditions compared to the reference zone. Despite being at the same distance from the green wall, participants reported feeling more thermally comfortable when facing the green wall than when sitting against it and viewing the same scene as in the reference space. This outcome might be attributed to visual effects, as humans tend to rely more on visual information for perception (Sinnett et al., 2007). Simply looking at a green wall could increase the level of thermal comfort.
Physiological responses measured using EEG, akin to questionnaire surveys, were influenced by different landscape amenities, each evoking distinct emotions. For instance, facing the green wall elicited the highest levels of engagement, relaxation, and interest compared to the reference. Sitting against the green wall resulted in higher excitement and focus levels. The greatest degree of stress was recorded under the shaded canopy.
When facing the green wall, all indices except stress were significantly higher compared to those measured in the reference zone, with engagement, relaxation, and interest particularly elevated. The well-established positive link between exposure to greenery and mental well-being supports these findings (Qin et al., 2021). Views of the natural environment are known to induce relaxation (Elsadek et al., 2020), while higher engagement and interest may stem from visual stimulation. Despite the smaller overall difference from the reference value compared to other greenery options, relaxation and interest levels remained higher than those observed under the shade canopy. Under the shade canopy, engagement, stress, interest, and focus were rated significantly higher than under the reference. Compared to the spatial setting with the facing-green-wall, which had the most positive influence on subjects’ emotions, every index showed a smaller difference from the reference setting, except for focus. Notably, stress levels were highest compared to all other spatial settings. This heightened stress may have been influenced by noise from the waiting area. The proximity of the shade canopy to the bench where participants waited for their turn could have led to increased stress due to noise from other participants, consequently reducing comfort levels (Nitidara et al., 2022).
Unlike other settings, the green wall presents a prominent object at eye level, likely prompting participants to focus on and contemplate it. The results when sitting against the green wall mirrored those of the previous setting, indicating higher levels of engagement, excitement, relaxation, interest, and focus. However, in this scenario, excitement and focus were particularly heightened among the spatial settings, with stress also elevated compared to the reference. This increased excitement and focus align with previous research demonstrating that exposure to greenery can enhance attention and performance in educational or work environments (Sadick and Kamardeen, 2020). Studies have shown improved cognitive performance and attention in classrooms with green walls, supporting these findings (Bernardo et al., 2021). When looking at the green roof, stress, relaxation, interest, and focus were rated higher compared to the reference, but the difference in magnitude was generally smaller than in other settings. This limited impact may be attributed to two factors: the small size of the green roof module and the choice of plant species, Sedum takesimense Nakai, selected more for practical reasons than aesthetic appeal (Tsantopoulos et al., 2018). Previous studies have suggested that green scenery can evoke varied emotional responses depending on its look; for instance, it has been suggested that ivy is more aesthetically pleasing than Sedum (White and Gatersleben, 2011).
It should also be noted that among the five spatial settings, all spaces with greenery systems, except those facing the green wall, exhibited higher stress values compared to the reference; however, the stress level when sitting against the green wall was not statistically significant. Given that internal or external stimulation perceived by humans can evoke stress (Lipowski, 2013), participants might experience higher stress levels in spaces with greenery systems compared to the empty reference space. While high levels of stress can have long-term negative effects on physiological and psychological health (Folkman, 2008), low-to-moderate levels of stress, known as positive stress, can improve arousal and productivity. Positive stress is typically infrequent, short-lived, and mild, and can also stimulate motivation and resilience (Franke, 2014).
4. Discussion and Conclusion
In this study, we explored the impact of landscape amenities on human thermal comfort across five outdoor settings: baseline, facing a green wall, sitting against the green wall, looking at the green roof, and being under the shade canopy. Using a multilevel model, we analyzed both physiological (EEG) and psychological (thermal sensation vote) data. This marks the first investigation into outdoor landscape amenity effects on thermal comfort utilizing EEG and questionnaire surveys simultaneously. Recognizing the influence of emotions on thermal comfort, we assessed both physiological and psychological states.
This study reveals a nuanced relationship between TSV and EEG indicators. While TSV results under the shade canopy demonstrated the highest thermal comfort, EEG data indicated elevated stress levels, likely due to external noise from the experimental setup. Conversely, greenery systems such as the green wall elicited higher engagement and relaxation in EEG data, even though TSV results showed lower thermal comfort compared to the shade canopy. These findings suggest that thermal comfort is influenced not only by physiological conditions but also by psychological factors, including visual and auditory stimuli. Integrating both measures provides a comprehensive understanding of how outdoor landscape amenities impact human well-being.
Unlike prior EEG studies confined to indoor spaces, our research took place outdoors, allowing for an evaluation of the real impact of landscape amenities. Survey results affirmed that people find greater thermal comfort under shaded canopies compared to greenery systems. Shading structures, such as canopies, directly block shortwave radiation, leading to immediate cooling effects in outdoor environments. By reducing solar radiation exposure, they significantly enhance thermal conditions for users (Xu et al., 2019). While shade provides superior cooling effects over evapotranspiration from greenery systems like green walls and roofs, the visual appeal of greenery systems positively influences perceived thermal comfort levels. Greenery systems improve thermal environments primarily through evapotranspiration, a process where plants release moisture into the air, thereby cooling the surrounding area (Jaafar et al., 2022). Additionally, vertical greening provides visual insulation from heat-intensive surroundings, creating a psychological perception of cooler conditions. Our findings support the notion that providing shade effectively enhances outdoor thermal conditions by reducing sunlight exposure, as indicated by previous studies (Xu et al., 2019). The questionnaire results reinforced this, showing that the shade canopy offered the most effective cooling among the strategies studied. However, EEG data revealed that participants experienced higher stress levels under the canopy, possibly due to noise from the nearby waiting area.
Consistent with prior research linking greenery to mental well-being (Cox et al., 2017), participants reported more positive emotions with greenery systems compared to the shade canopy. Vertical greenery, such as the green wall, was particularly effective in reducing stress, while the spatial setting with a green roof induced higher relaxation levels than under the shade canopy. Although statistically insignificant compared to the reference, the presence of greenery systems led to improved thermal comfort and psychological well-being. The visual impact of greenery, especially vertical greenery like green walls, enhances users’ thermal comfort perception by creating a calming environment that reduces stress and increases relaxation (Elsadek et al., 2020). This aligns with findings that the greenery’s visual stimulation aids in psychological cooling, even without significant temperature changes. This effect may be attributed to visual cues from the plant material (Elsadek et al., 2020), as the stress-alleviating effect was less pronounced when participants sat against the greenery system.
The practical implications of this study are as follows: Improving outdoor thermal environments aims to create pleasant urban spaces for residents. Natural green spaces, including small features like green walls and roofs, offer positive psychological effects by supporting mental health. In light of these findings, incorporating small-scale greenery systems into urban planning can significantly improve environmental quality, particularly in densely populated areas where access to larger green spaces is limited. For example, green walls can be strategically installed in areas with high pedestrian activity to maximize their visual and psychological benefits. Moreover, green roofs may be particularly useful in urban rooftops of commercial or residential buildings, contributing to overall thermal regulation and offering visual relief to nearby residents.
Despite higher upfront costs associated with green roofs and walls, including plant procurement, irrigation, and maintenance expenses (Nordman et al., 2018), the long-term benefits, including enhanced mental well-being and improved thermal comfort, justify their integration into urban infrastructure. These systems are not only effective in mitigating urban heat but also serve as a sustainable alternative to conventional shading solutions, such as canopies, by providing additional ecosystem services. To maximize impact, future urban policies should prioritize the visibility and accessibility of greenery systems. Integrating greenery into urban design guidelines will ensure its implementation at scales and locations where its benefits are most pronounced.
This study encountered certain limitations that should be acknowledged. Firstly, it did not employ standard randomization procedures, which are essential for minimizing bias and ensuring the validity of study results. While less critical in repeated measures designs, randomizing conditions’ order or counterbalancing sequences across participants helps mitigate potential order effects and maintain result integrity. Additionally, the experimental site, particularly the green roof, may have lacked sufficient scale to provide participants with a comprehensive environmental experience. Despite referencing previous studies to determine greenery system size, our installations remained relatively small compared to typical urban landscape amenities. While the greenery systems, such as the green wall measuring 2200 × 2300 mm, were sufficient to offer participants a clear visual effect, their relatively modest scale could limit the extent to which real-world conditions are reflected. Given that greenery size and quality impact both cooling effects and user perceptions, this limitation may have dampened physiological responses, diminishing the full potential of greening effects.
The reference setting afforded participants a wide vision, potentially inducing psychological effects. As the experiment occurred outdoors, external disturbances like airplane noise were uncontrollable. The study also had a relatively small number of participants, with 75.4% of them in their 20s, which limits the generalizability of the findings. Future studies should aim to recruit a more diverse participant pool in terms of age and demographics to ensure broader applicability of results. By recognizing these limitations, we aim to provide a comprehensive understanding of constraints and encourage further research to address them and enhance future findings’ validity.
Our result revealed higher stress levels under landscape amenities compared to the reference, except when facing the green wall. Regarding the EEG data, while higher indices of engagement and relaxation were observed under greenery systems, it is important to exercise caution in concluding that “greenery systems induce mental relaxation.” EEG data can be influenced by various complex factors, including the environment and individual differences. Thus, such conclusions should be presented as tentative, with additional experiments suggested for further validation. More sophisticated methods, including elaborate mental states questionnaires, could improve understanding of the relationship between EEG responses and mental states in future research. Moreover, our results underscore the visual impact of greenery systems on thermal alleviation. Further investigations could assess whether artificial greening yields similar thermal comfort improvements. Additionally, expanding the sample size and including participants from various age groups will help validate and refine the findings, addressing current study limitations. These findings advocate for the integration of outdoor landscape amenities to enhance summer environmental quality. Policymakers can use our findings to inform decisions regarding the implementation of greenery systems for thermally pleasant outdoor environments.